The Giant Magellan Telescope is easily the most ambitious terrestrial astronomy program humanity’s ever devised. It has — quite literally — been built from the ground up by leveraging a brilliant, unique off-axis design and bleeding-edge fabrication techniques. And nowhere is that more apparent than in the telescope’s enormous mirror system.
These mirrors, which are still in the process of being cast, ground, and polished to immensely tight optical tolerances, are the result of a revolutionary production process developed by the team at University of Arizona’s Steward Observatory Mirror Lab. We sat down with Buddy Martin, an astronomer and U of A Project Scientist who has headed up the university’s optical polishing program since the 1980s, for more on how these incredible mirrors are being made.
Gizmodo: Can you tell me a bit more about the mirrors themselves — where is the glass sourced from, what does the production process entail?
We buy the glass from Ohara, a Japanese company. It’s a high-quality borosilicate glass very similar to Pyrex — at Ohara, they call it E-6 glass.
Some basic facts about the mirrors:
- They’re the largest mirrors ever made
- They’re the only large lightwave mirrors ever made
- They’re arranged in a honeycomb structure that makes them 5x lighter than a solid mirror of the same dimensions.
This imparts a number of advantages, which is why the GMTO decided to use these mirrors. They remain stiff thanks to the honeycomb structure and won’t distort under its own weight nearly as much as other mirror designs would. And because all the glass sections are very thin — nothing is more than 28 mm thick — the glass follows the temperature of the air around it so as the mirror cools through the night, the glass cools with it. And that avoids creating turbulence that could spoil the images.
For the GMT, that means you can make a 25 m telescope with very few moving parts, or parts that you have to control — it’s only got the seven primary mirror segments and the seven secondary mirror segments.
And among the competing projects, the next simplest had more than 500 primary mirror segments (that’s the 30 m telescope) and the European project had even more than that: 700 – 800 segments. It makes it so there are fewer pieces that you have to control at a high accuracy.
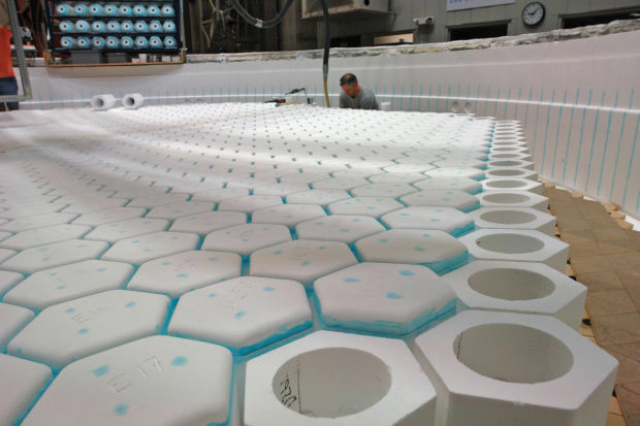
The honeycomb-shaped substrate of the mirrors. Image: University of Arizona
And how are these mirrors coated and polished?
The polishing is an interesting problem because of the shape of these mirrors — they’re very very different from a spherical surface that has a constant curvature. And that’s kind of been the history of optics fabrication — how to make more and more aspheric curvatures.
The first telescope that Isaac Newton made had to have a spherical mirror because that was the only thing they could produce at the time. And he could get away with it because it was a very small telescope. But with larger telescopes you have to make the mirror a more parabolic shape and when you get up to 25 meters there’s a big difference between that parabolic shape and a spherical shape.
With the GMT, we’re dealing with 14 mm of deviation from a spherical shape — it looks like a saddle, or a Pringles chip. It’s really challenging to polish that shape and even more challenging to measure it accurately. Almost everything we do has a natural tendency to work with a spherical surface where the curvature is uniform and we have to essentially force it to work with this strange parabolic shape — every tool has to accommodate this changing curvature.
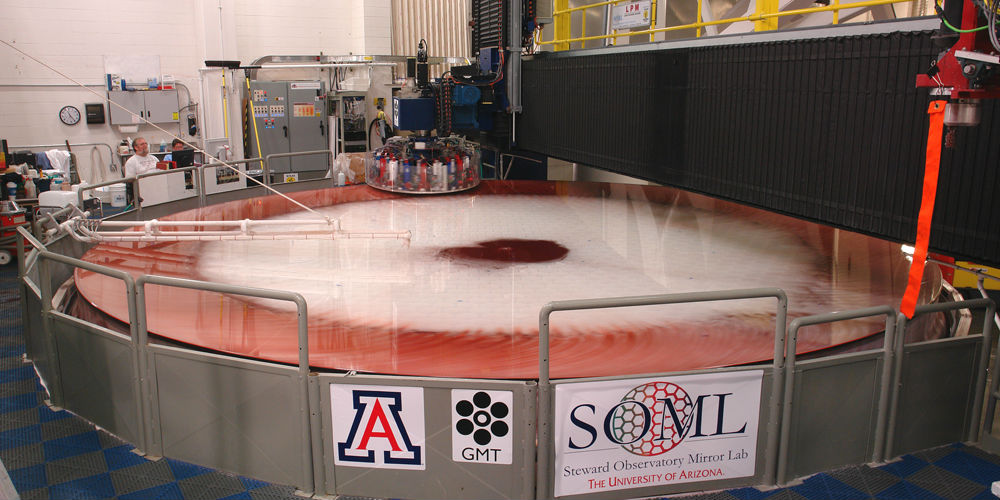
Image: Ray Bertram, Steward Observatory, University of Arizona
We use a variety of tools, primarily of two types: we have an active tool called a stress lap — a lap is just a general name for a polishing tool that we stretch, or “stress” across the mirror surface to match its curvature at any given instant. That’s a technology that we developed here in order to make more aspheric surfaces, which is what the telescope designs were calling for. It’s a relatively large tool — about a meter in diameter — and it’s very stiff so that if you rub it on a mirror surface, it’s stiff enough to naturally “pick out” the high spots, the parts that need to be worn down, and makes it smoother.
The other type of tool we use is a smaller, more pliant tool — it’s flexible enough to follow the changing curvature of the mirror — those are between about 10 and 35 cm in diameter. We use those, not for smoothing — they’re not stiff enough for that — but rather for “direct disfiguring” where we identify the high spots and spend more time rubbing on those spots to bring them down to match the lower places.
It’s a very iterative process. we got through 50 to 80 cycles of polishing and measuring. When we measure, we generate a contour map of the errors in the mirror surface. We feed those maps into a computer that control the polishing tools so that it either spends more time or exerts more pressure on the high spots.
Wait, so the process is automated?
It’s largely automated yes. At least the polishing itself is. When we’re running these tools around on the glass, it’s completely computer controlled. The human element is deciding what kind of tactics to use based on the error map.
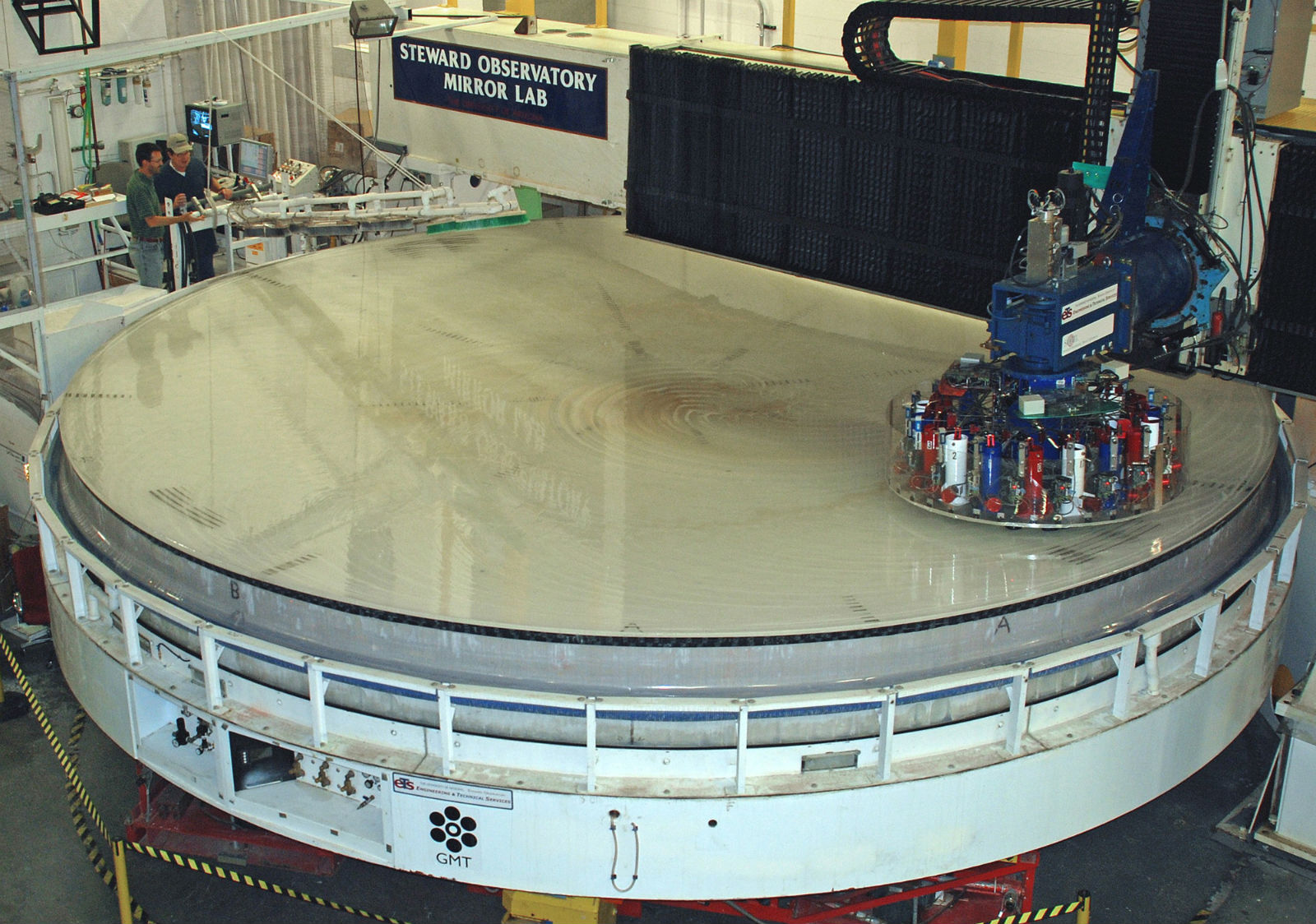
Image: Carnegie Institution for Science
And what do you use to generate that contour map?
There are a number of different measuring devices. The main and most important one, and this is pretty much standard throughout the industry, is called an interferometer. It illuminates the mirror surface with laser light, the light reflects back to the instrument which collects that light.
The optics in the device are set up so that with a perfect mirror, the light will return with the same wavefront that initially left the instrument which is recognised as a perfect surface.
If the mirror has a bump on it, then that gets imprinted onto the returning wavefront and the instrument can analyse that light coming back, identifying the highs and lows to a resolution of about 1/100th of a wavelength — about 5 nm.
And what sort of accuracy tolerances is the team aiming for?
Roughly speaking, we can have an average error of about 25 nm. That is, the surface has to be within 25 nm — one millionth of an inch — of a “perfect” surface shape. The measurements (which, again, resolve down to 5 nm) are good enough, the bigger challenge is polishing it and controlling the surface at a 25 nm accuracy.
How long does it take to fully grind and polish a mirror segment?
It’s roughly a year of polishing per mirror. We’ve only finished one, and that was the first. It was the first mirror of this kind that had ever been built anywhere so it took us a little longer with that one as we had to develop and build all of the equipment and techniques for it. We’re already beginning work on the second mirror and we’re expecting that, once we hit our stride, it will be a year of polishing per segment.
There are other stages of the manufacturing too. First we have to melt the glass into its form, then we have to do some grinding. Those are more or less the three stages: casting the mirrors, grinding them into shape, then polishing them. Fortunately, we have three stations so we can be working on three mirrors at the same time.
So how much deviation do the mirrors exit the casting process with? How much grinding is required before the mirror goes on to the polishing stage?
It’s worth noting that we do spin casting so we’re rotating the glass as it’s melted which will eventually form a parabolic surface but not one that’s terribly accurate. We’re not trying to achieve optical quality here. For a more traditional, symmetric mirror, we can get it within about a millimetre of the right shape out of casting. But because of uncertainties in the casting process we also have to add some extra glass so it ends up being a 6 to 8 mm deviation that we have to grind out.
Now GMT mirrors are different in that they have these 14 mm of saddle shape departure from a perfectly symmetric surface so our casting process — if you spin a liquid, you get a parabola and that’s what our casing produces. We have a symmetric parabola which is the best approximation of the saddle-shaped, off-axis parabola that we need so we always have to grind in that 14 mm of saddle shape.
The mirror has that shape is because the entire 25 m telescope is a symmetric parabolic mirror — but the segments, which are not in the center, hence “off-axis” — are asymmetric pieces of that larger parabola and when you take these offset pieces of it, you see the 14 mm of saddle-shaped departure. That’s what we need to put into the outer segments of the GMT mirror. During grinding, we get down to an accuracy of about 100 microns. Then the polishing will reduce that gradually down to the 25 nm tolerance.
Do you foresee any other applications for these polishing tools and methodology outside of the GMT project?
It’s a fairly specialised process but is good for more than just large astronomy telescope mirrors. Really any sort of precise optical system that uses lenses or mirrors — especially aspheric varieties — can benefit from this. The technology is being used in other places to make mirrors for telescopes, imaging systems, and light-focusing optics for fusion energy programs.